The shipping industry contributes 3% of global emissions, responsible for approximately 900 million tons of CO2 emissions worldwide. The International Maritime Organization (IMO) established its Initial IMO GHG Strategy back in 2018, with aims to reduce the carbon intensity of international shipping by at least 40% by 2030 and pursue efforts towards 70% by 2050. Additionally, the goal was to reduce total annual GHG emissions from international shipping by at least 50% by 2050, compared to 2008 levels.
Two significant actions were taken to achieve these goals. The “IMO 2020 0.50% sulfur limit” was enforced in 2020, aiming to reduce sulfur oxide emissions from shipping by over 75%. In 2021, short-term measures to decrease the carbon intensity of all ships by 40% by 2030, compared to 2008, were also enforced. These measures require new ships built from 2022 to be significantly more energy-efficient than the baseline.
Currently, nearly 240 stakeholders in the shipping industry, including ship owners, associations, ship builders, port and terminal operators, charterers, insurers, and more, have called upon governments to take action for shipping decarbonization. They are advocating for a zero-emission target for international shipping by 2050, support for zero-emission shipping projects, and policy measures to make zero-emission shipping the default choice by 2030. In response to the growing awareness, after the CoP26 event in November 2021, IMO’s Marine Environment Protection Committee (MEPC) decided to expedite the revision and strengthening of the Initial Strategy by June 2022.
Shipowners are already placing orders for zero-emission vessels set to be operational by 2024. Investors, banks, and insurance companies are demanding net-zero roadmaps from projects, while some major cargo owners and customers are insisting on zero-emission shipping for their entire goods by as early as 2040. The “First Movers Coalition for hard-to-abate sectors,” led by the US Presidency, has committed to shipping 10% of cargo using zero-emission fuels by 2030 and converting 5% of shipping fuel to zero-emission by 2030. The “Getting to Zero Coalition” has proposed “Green Shipping Corridors” suggesting that high-volume and high-carbon emission maritime routes, such as the Australia-Japan iron ore route and the Asia-Europe container route, be converted to zero-emission shipping.
However, the path to achieving zero-emission vessels is not yet clear, and decisions need to be made today to prepare for 2030 and beyond, considering that vessels have long lifespans (typically 25-35 years). Therefore, the industry must soon embrace one or more low-carbon fuel solutions.
The following fuels are under discussion:
Ammonia (NH3):
Ammonia, whether produced using hydrogen from renewable energy (“green” ammonia) or hydrogen from carbon capture and storage (CCS) (“blue” ammonia), appears to be a promising candidate for scaling up due to its high energy density, mature production technology, existing infrastructure, and carbon-free molecular composition. However, the high toxicity of ammonia poses a significant concern in case of a leak. To use ammonia as a maritime fuel, specialized safety standards, leakage safety measures, and port and bunkering infrastructure are necessary. As of now, no commercially available engines can use ammonia, but the first engines are expected to enter the market by 2025.
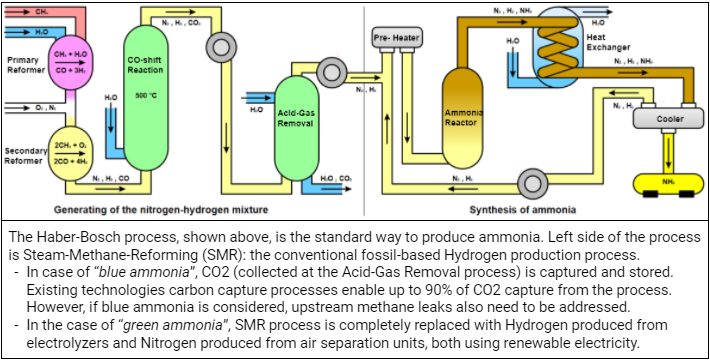
Methanol (MeOH):
Methanol is currently produced, stored, and traded on a large scale, similar to ammonia. Like ammonia, its existing production methods can be decarbonized by using low-carbon hydrogen. Moreover, methanol has lower toxicity and is environmentally degradable in case of a leakage. Both methanol engines and vessels are technologically ready, with some already in use. However, the main drawback of methanol is its carbon content and associated CO2 emissions during use. To be a genuine net-zero fuel alternative, the carbon in methanol must come from bioenergy with carbon capture (BECCS) or direct-air carbon capture and storage (DACCS). BECCS faces scalability concerns in terms of biomass availability and carbon capture technology costs. DACCS is an immature technology that is not cost-competitive at present.
Alternatively, carbon capture and utilization (CCU) from fossil-based production can be used to source the carbon in methanol. In such a case, it would be considered a delayed-emission rather than a zero-emission fuel, subject to environmental criticism. Nevertheless, for some fossil-based industries expected to continue after 2050 using carbon capture, utilizing the carbon in marine fuel could be a legitimate solution, especially when sequestration may not be practically feasible.
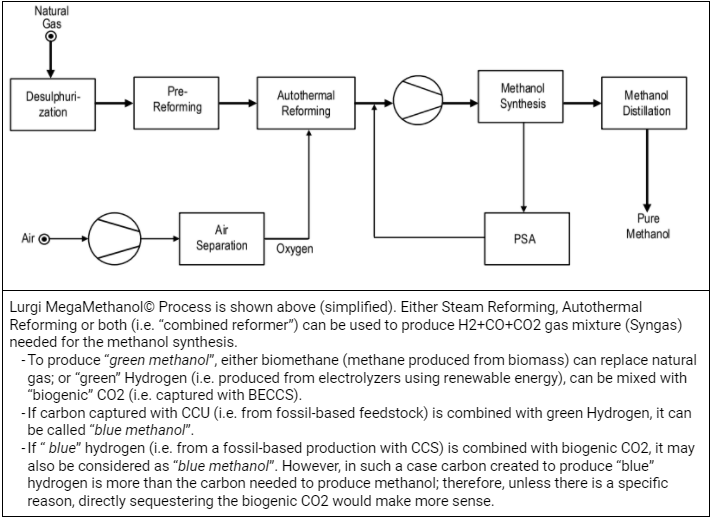
Liquid Hydrogen (LH2):
Hydrogen can serve as a direct energy source in the future. While hydrogen boasts high mass energy density (thanks to being the lightest element in the universe), its volumetric energy density is relatively low, necessitating cryogenic storage below -253 ºC—a significant vessel design challenge. Hydrogen liquefaction increases costs and limits infrastructure development and bunkering in ports.
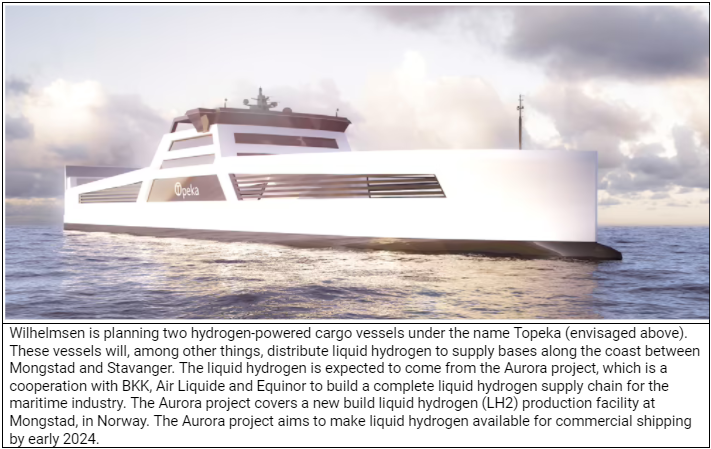
Biofuels
Biomass-based drop-in liquid fuels offer an easy adaptation opportunity, requiring minimal or no modifications to existing ship and engine designs. However, the long-term scalability and high production costs of biofuels pose major questions. Even if a sufficient supply of advanced biomass were globally available (from organic waste streams of industry and agriculture and woody residues from forest harvesting), sustainable fuel production for hard-to-abate sectors such as aviation might be better suited to utilizing these feedstocks.
In terms of costs, a study conducted with support from McKinsey predicts that by 2030, the Total Cost of Ownership (TCO), including capex, opex, and opportunity cost for lost cargo space in the case of larger volume zero-emission fuels, will have a gap of approximately 24 million USD for MeOH, LH2, and biofuels, while ammonia is somewhat 20% lower. By 2050, the TCO gap for each of these fuels is expected to decrease by 2-3 million USD.
Wind Power
Wind power, a historical maritime “fuel,” is gaining attention again in the quest for sustainable solutions. Various types of sails, including rotor sails (utilizing the spinning force of the Magnus effect for propulsion), telescopic or solid wind sails, and wing sails, are being implemented to reduce fuel consumption. However, wind power is viewed more as a supporting solution to reduce overall fuel consumption rather than a primary solution.
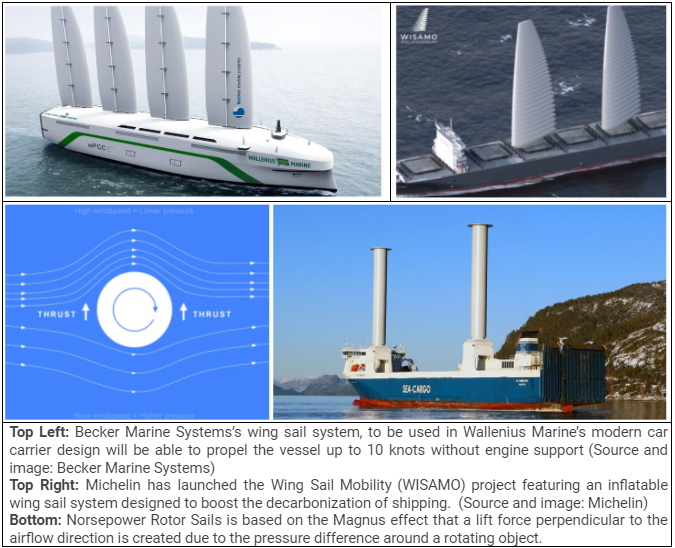
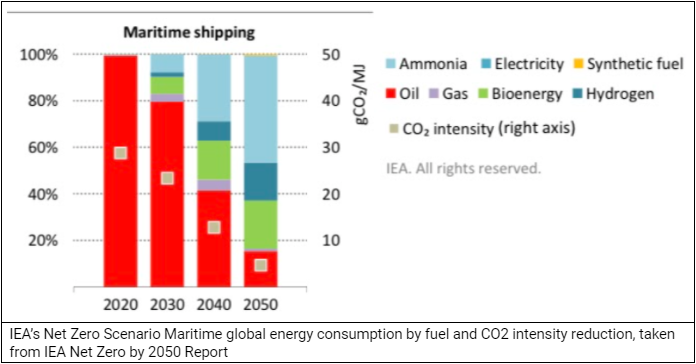
Among these options, the International Energy Agency expects ammonia to lead the way, followed by green methanol and hydrogen. However, as technologies develop concurrently, it will be fascinating to observe which technologies gain widespread adoption in the coming years.
Top Credit: Energy Observer, the first hydrogen vessel around the world, explores concrete solutions and develops technologies to accelerate ecological transition by testing hydrogen technologies in extreme environments. Since its launch in April 2017 in Saint-Malo, it has sailed fully autonomously for 4 years over 75,000 km in extreme conditions, through the Mediterranean, then the Arctic and the Atlantic, and in the Pacific at the time this article was written.
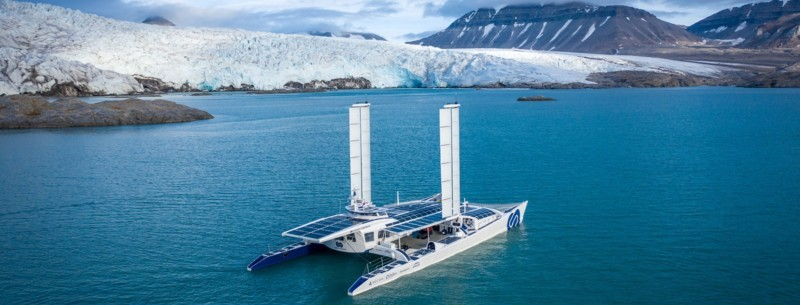
Edit in Feb-2022:
Energy Observer-2 is following the foot steps of the Energy Observer to demonstrate a multipurpose cargo ship fuelled by liquid hydrogen.
Its specifications have been established to replace multipurpose cargo ships of about 5 000 tons deadweight which constitute nearly 37% of the world fleet, often with old and polluting designs.
Photo credits: Kaddd & Epron Design
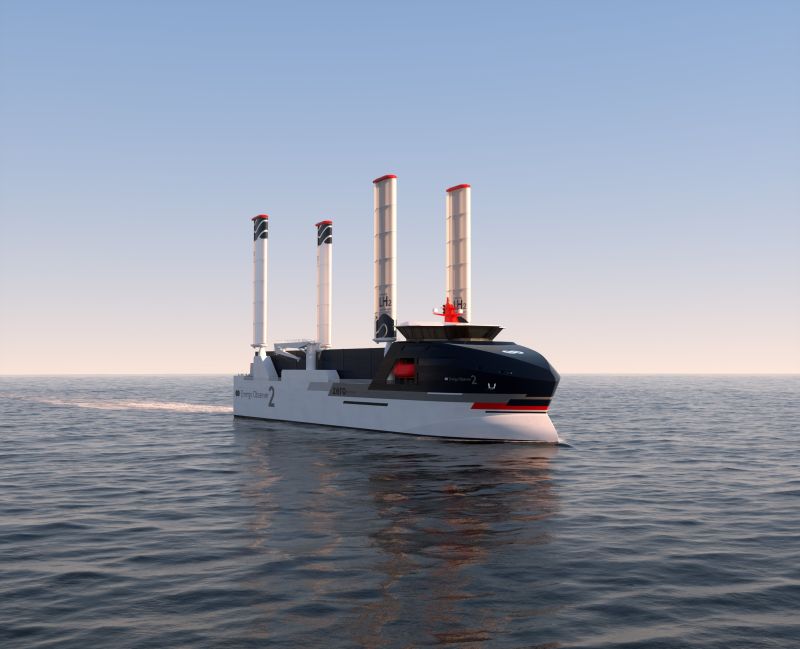
The main features of Energy Observer 2 are:
Length: 120 meters
Width: 22 meters
Draft: 5,5 meters
The surface of the wings: 1450 m²
Deadweight: 5,000 tons
Containers: 240 TEU
Ro-ro bridge: 480 linear meters
Tween deck height: 6.5 meters
Access ramp: 15 meters wide
Commercial speed: 12 knots
Electric propulsion: 4 MW
Fuel cell power: 2.5 MW
Liquid hydrogen tanks (LH2): 70 t
Range: up to 4,000 nautical miles